Enzyme structure-function relationships
Enzyme Discovery
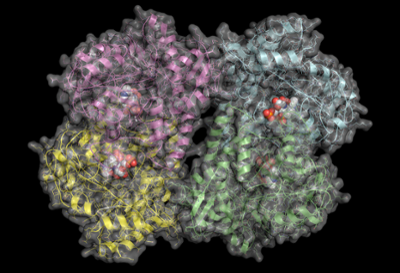
There are many enzymes whose functions are unknown or poorly understood. We approach such problems in the context of enzyme superfamilies, which are groups of enzymes that have evolved with considerable conservation of structure and chemical mechanism. This allows us to predict function and investigate catalytic activities. This will often require the synthesis of proposed substrates, and the acquisition of high-resolution crystal structures at the Canadian Light Source. We frequently collaborate with Dr. David Sanders on such projects. Our primary interest is in enzymes related to myo-inositol, carbohydrate-active enzymes, and enzymes required for antibiotic biosynthesis.
Example publications on enzyme discovery:
- Prasertanan et al., The kanosamine biosynthetic pathway in Bacillus cereus UW85: functional and kinetic characterization of KabA, KabB, and KabC. Archives of Biochemistry and Biophysics, 2019, 676, 108139. link
- Aamudalapalli et al., myo-Inositol dehydrogenase and scyllo-inositol dehydrogenase from Lactobacillus casei BL23 bind their substrates in very different orientations. BBA - Proteins and Proteomics, 2018, 1866, 1115 - 1124. link
- Vetter et al., A Previously-Unrecognized Kanosamine Biosynthesis Pathway in Bacillus subtilis. Journal of the American Chemical Society, 2013, 135, 5970 - 5973. link
- Phenix et al. Structural, functional and calorimetric investigation of MosA, a dihydrodipicolinate synthase from Sinorhizobium meliloti L5-30, does not support involvement in rhizopine biosynthesis. ChemBioChem, 2008, 9, 1591 - 1602. link
Mechanistic enzymology
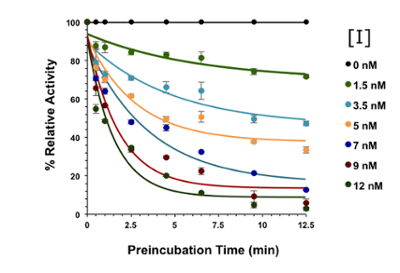
For enzymes to achieve enormous rate enhancements, they use a collection of chemical strategies to overcome reaction barriers. An inhibitor binds to an enzyme to modulate catalysis, but these effects are often complex. Understanding the mechanism of an enzyme is the key to understanding its potential as a catalyst, and the most effective and selective way to target that enzyme for inhibition. We use steady-state kinetics approaches to examine the chemical and kinetic mechansisms of enzymes of interest, using techniques such as UV-visible spectroscopy, fluorescence, HPLC, and NMR to monitor the reaction.
Example publications on mechanistic enzymology:
- Vetter & Palmer, Simultaneous measurement of glucose-6-phosphate 3-dehydrogenase (NtdC) catalysis and the nonenzymatic reaction of its product: kinetics and isotope effects on the first step in kanosamine biosynthesis. Biochemistry, 2017, 56, 2001 - 2009. link
- Zheng et al., Converting NAD-specific inositol dehydrogenase to an efficient NADP-selective catalyst, with a surprising twist. Biochemistry, 2013, 52, 5876 - 5883. link
- Skovpen & Palmer, Dihydrodipicolinate synthase from Campylobacter jejuni: kinetic mechanism of cooperative allosteric inhibition and inhibitor-induced substrate cooperativity. Biochemistry, 2013, 52, 5454 - 5462. link
Inhibitor Design and Synthesis
Antibiotic targets
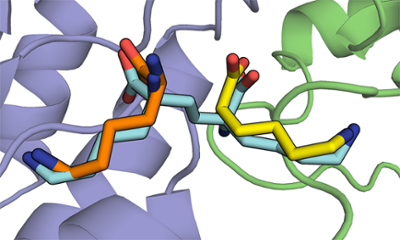
There are many enzymes that are essential for bacterial growth, but are not found in other organisms, such as humans. Compounds that can inhibit such enzymes selectively may act as antibiotics, such as penicillin. Screening large libraries of compounds has resulted in many useful drugs, but has not been a successful approach in antibiotic discovery. Designing inhibitors requires a knowledge of either the enzyme's catalytic mechanism, high-resolution structure, or both.
We design inhibitors of putative antibiotic targets in order to understand these enzymes better, and generate leads for new avenues of medicinal chemistry. We have had success with both active-site directed competitive inhibitors, and allosteric inhibitors. This requires skill in both organic synthesis and kinetic methods.
Example publications on inhibitors of antibiotic targets:
- Skovpen et al., Biomimetic design results in a potent inhibitor of dihydrodipicolinate synthase from Campylobacter jejuni. Journal of the American Chemical Society, 2016, 138, 2014 - 2020. link
- Fang, et al., Succinylphosphonate esters are competitive inhibitors of MenD that show active-site discrimination between homologous alpha-ketoglutarate-decarboxylating enzymes. Biochemistry, 2010, 49, 2672 - 2679. link
Active-site probes
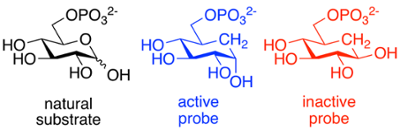
We use organic synthesis to prepare compounds that help us understand an enzymatic reaction and its mechanism. If an inhibitor-enzyme complex can be crystallized, we can identify key residues and predict their roles in catalysis.
Probes can also be used as selective means of identifying and labeling enzymes; for example, a fluorescent "tag" or radiolabel can allow these enzymes to be located in cells and tissues. They may also stabilize the protein, and act as a "molecular chaperone". We work in collaboration with Dr. Chris Phenix and others on such projects.
Example publications on active-site probes:
- Vetter & Palmer, Substrate substitution in kanosamine biosynthesis using phosphonates and phosphite rescue. Biochemistry, 2021, 60, 1926–1932. link
- Vetter et al., Carbocyclic substrate analogs reveal kanosamine biosynthesis begins with the alpha-anomer of glucose 6-phosphate. ACS Chemical Biology, 2020, 15, 2205 - 2211. link
- Fang et al., Using substrate analogues to probe the kinetic mechanism and active site of Escherichia coli MenD. Biochemistry, 2011, 50, 8712 - 8721. link
Biocatalysis
Enzymes in organic synthesis
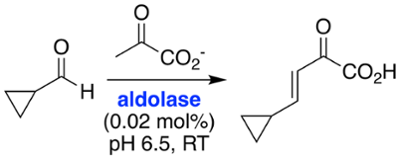
Enzymes can be applied to the preparative-scale synthesis of compounds of interest. This is known as biocatalysis, and has advantages over conventional synthesis because enzymes are typically very selective catalysts, often displaying high stereoselectivity. Biocatalysis can avoid the use of toxic solvents and metals, and can therefore be considered "green chemistry".
Biocatalysis is an emerging area of interest in our laboratory. We use our knowledge of organic chemistry and our understanding of enzyme superfamilies to find new and useful transformations and to generate compounds of interest.
Example publications on biocatalysis:
- Fansher and Palmer, A Type 1 Aldolase, NahE, Catalyzes a Stereoselective Nitro-Michael Reaction: Synthesis of β-Aryl-γ-nitrobutyric Acids. Angewandte Chemie - International Edition, 62, e202214539. link
- Fansher et al., Repurposing an aldolase for the chemoenzymatic synthesis of substituted quinolines. ACS Catalysis, 2021, 11, 6939–6943. link
- Ramos-Figueroa et al., Preparation and application of 13C-labeled myo-inositol to identify new catabolic products in inositol metabolism in Lactobacillus casei. Biochemistry, 2020, 59, 2974 - 2985. link