Our Research
What are Quantum Materials?
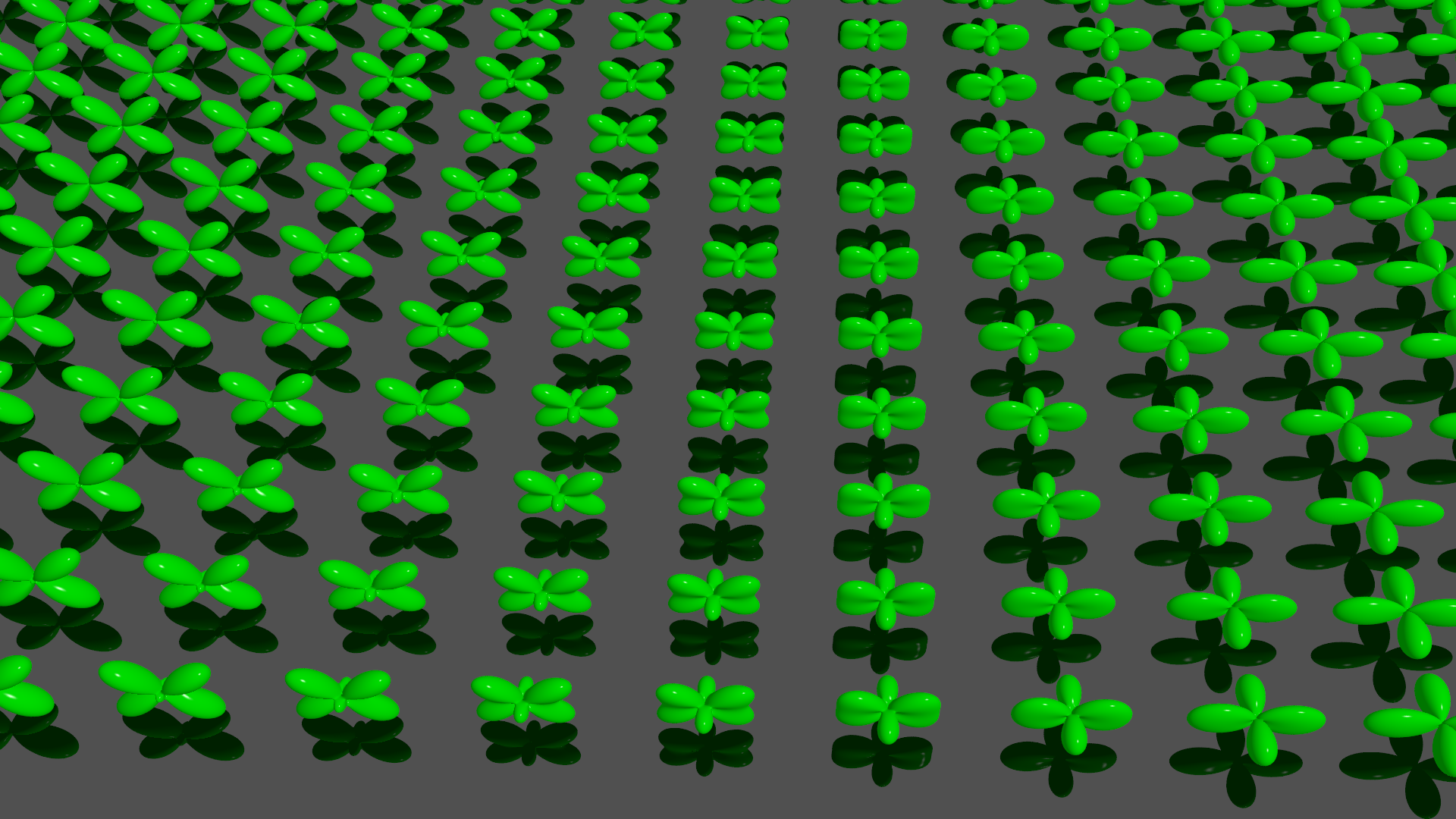
At the atomic level, all materials are quantum in the sense that the electrons and nuclei behave according to the principles of quantum mechanics. However, the term Quantum Materials has emerged in recent years to refer to materials exhibiting any of a broad range of properties including electron correlations, electronic order (spin, charge, or orbital), superconductivity, or other properties which are specifically quantum in nature.
Quantum materials have become highly studied in recent years, due to potential groundbreaking applications in various industries such as consumer electronics, telecommunications, renewable energy, and next-generation computing.
Transition Metal Oxides: Correlations, magnetism, and more!
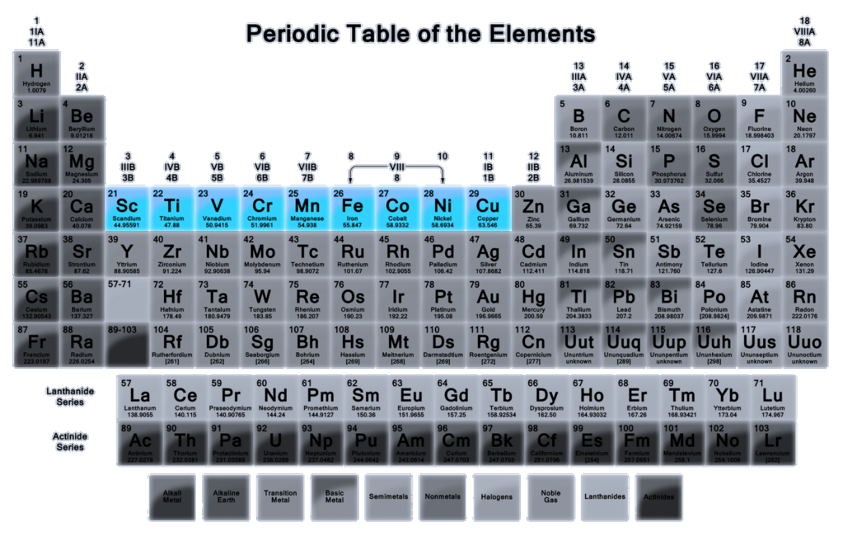
One of the major classes of materials we study are oxides containing transition metals from the 3d row of the periodic table. Over the past century, these transition metal oxides have continually surprised physicists with unique and unexpected properties: from puzzling insulating behaviour which challenged early band theory in the 1930s, to the discovery of high temperature superconductivity in the 1980s (which remains unexplained today), this family of materials repeatedly tests our understanding of condensed matter physics.
In particular, oxides having the perovskite structure (ABO3) show many fascinating properties: temperature dependent metal-insulator transitions, various forms of (anti)ferromagnetism, high temperature superconductivity, and more. The perovskite stoichiometry pushes the transition metal atoms to relatively high oxidation states, causing the electrons to straddle the line between localized and itinerant physics, leading to these diverse properties.
Oxide Heterostructures: Designing materials, atom by atom
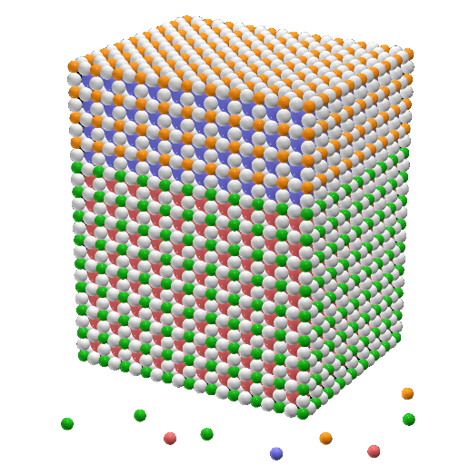
While the bulk forms of the 3d transition metal oxides exhibit a wide range of fascinating properties, now an entire new set of properties is emerging via the field of oxide heterostructures. Researchers use advanced film growth techniques to grow multilayer films with atomically abrupt interfaces. These interfaces between transition metal oxides are often found to exhibit phenomena entirely different from the corresponding bulk materials. This includes high-mobility 2D electron liquids, ferromagnetism between non-magnetic materials, and even superconductivity at interfaces between robust insulators. Such findings are very exciting from an applications viewpoint, but many questions remain about the origin and detailed characteristics of these often mysterious properties. Such nanometer-scale regions at buried interfaces are inherently difficult to study, which is where we enter with our resonant x-ray spectroscopy techniques.
Resonant X-ray Spectroscopy: Probing bulk, interfaces, and ordering phenomena
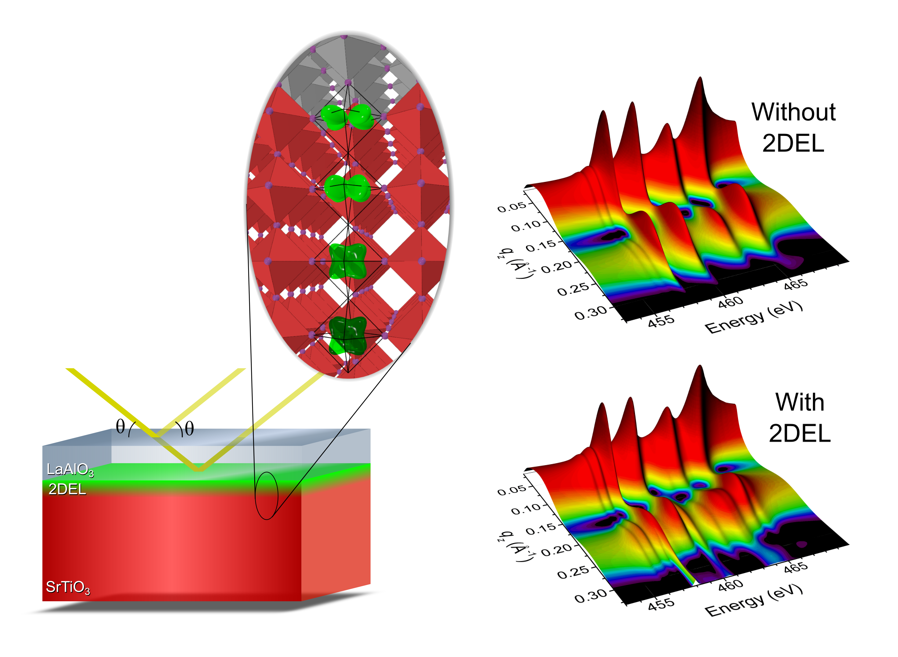
Our primary experimental tool for the study of quantum materials is synchrotron-based resonant x-ray spectroscopy. We illuminate our samples with x-rays having energies precisely tuned to core-level resonances, and observe how the x-rays are absorbed, diffracted, or reflected from the sample.
X-ray Absorption Spectroscopy is a technique which probes the bulk electronic structure of a material. A measurement which averages over the local atomic scattering tensors, XAS can probe the valences, spin states, and orbital occupations of atoms, providing key information about the behavior of electrons in the material.
Resonant X-ray Diffraction probes ordering phenomena in materials (such as magnetism, orbital order, and charge order).
Resonant X-ray Reflection is a new technique we use to probe depth dependence and interface effects in oxide heterostructures.
Theoretical Modelling: Pushing computation to the limit
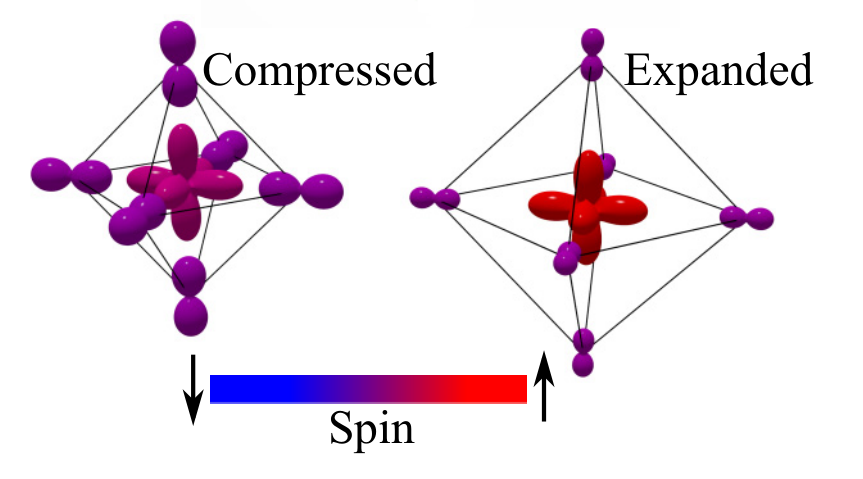
Our work on correlated electron systems often involves computation of quantum many-body models for the determination of local properties of materials and the computation of spectral functions. We use and actively contribute to the software Quanty, which performs exact diagonalization of quantum many-body models and uses a Green's function approach to compute x-ray spectral functions. One of our recent projects which extensively used Quanty to develop a new type of local model can be found here: Physical Review B 94, 195127 (2016). We additionally use the program QUAD to simulate the reflection and diffraction of resonant x-rays from heterostructure systems. A recent publication on the capabilities of QUAD can be found here: Physical Review Letters 117, 115501 (2016).