Research
Our Techniques
X-RAY EMISSION SPECTROSCOPY
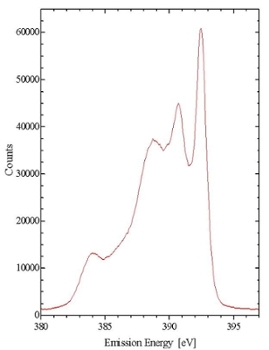
X-ray Emission Spectroscopy (XES) provides a means of probing the partial occupied density of electronic states of a material. XES is element-specific and site-specific, making it a powerful tool for determining detailed electronic properties of materials.
Emission spectroscopy can take the form of either resonant inelastic x-ray emission spectroscopy (RIXS) or non-resonant x-ray emission spectroscopy (NXES). Both spectroscopies involve the photonic promotion of a core level electron, and the measurement of the fluorescence that occurs as the electron relaxes into a lower-energy state. The differences between resonant and non-resonant excitation arise from the state of the atom before fluorescence occurs.
In resonant excitation the core electron is promoted to a bound state in the conduction band. Non-resonant excitation occurs when the incoming radiation promotes a core electron to the continuum. When a core hole is created in this way, it is possible for it to be refilled through one of several different decay paths. Each of the peaks in the Figure corresponds to a different decay path. Because the core hole is refilled from the sample’s high-energy free states, the decay and emission processes must be treated as separate dipole transitions. This is in contrast with RIXS, where the events are coupled, and must be treated as a single scattering process.
Soft x-rays have different optical properties than visible light and therefore experiments must take place in ultra high vacuum, where the photon beam is manipulated using special mirrors and diffraction gratings.
Gratings diffract each energy or wavelength present in the incoming radiation in a different direction. Grating monochromators allow the user the select the specific photon energy they wish to use to excite the sample. Diffraction gratings are also used in the spectrometer to analyze the photon energy of the radiation emitted by the sample.
X-RAY ABSORPTION SPECTROSCOPY
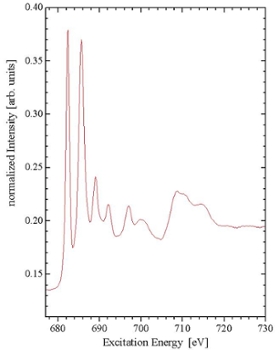
X-ray Absorption Spectroscopy (or XAS) involves observing the fraction of photons which are absorbed when monochromatic synchrotron radiation is incident upon a sample. The mechanism by which photons are absorbed is the promotion of core-level electrons into higher-energy unoccupied states. Therefore, XAS is a direct probe of the partial density of unoccupied electronic states within the sample, allowing direct probing of the conduction band.
To provide a picture of the density of unoccupied states within a certain energy range, the excitation energy is increased by fixed amounts, and the amount of energy absorbed is plotted as a function of the excitation energy. These plots have large, distinct features at energies corresponding to resonant excitation from one bound state to another. In this way, the energy levels of the unoccupied states (relative to the occupied states) can be determined.
There are several methods for measuring XAS. One method is total electron yield (TEY) measurements. TEY involves measuring the amount of electrons needed to neutralize the sample as electrons are ejected. The amount of photons absorbed through excitation to bound states can be measured, even though these excitations do not directly cause electrons to be ejected. In this case, measurements are made possible by the fact that the excited electron quickly relaxes into a lower-energy state, releasing energy. This energy is often transferred to a bound electron, which is then ejected from the sample, leaving a hole which must be refilled. This produces a net positive current out of the sample.
Although the ejection of electrons is the most probable decay mechanism, it is also possible for the energy to be released in the form of a photon. Absorption spectra created by detecting these photons are known as fluorescence yield measurements. Fluorescence methods have advantages over TEY measurements, in that they are not sensitive to sample charging, and have greater bulk-sensitivity. This is due to the fact that photons have a greater escape depth than electrons. Radiative transitions are governed by the selection rule describing the change in l = +/-1 (l is the orbital quantum number).
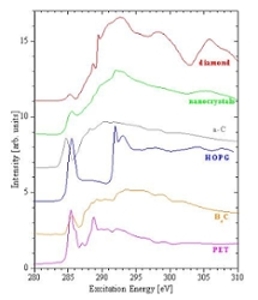