Theme 1
Isolation of bioactive natural products from plant pathogens: phytotoxins, elicitors and mycotoxins
Fungal pathogens of plants produce secondary metabolites that can damage the plant cells irreversibly, i.e. phytotoxins. Some phytotoxins cause plant disease symptoms similar to the pathogen and affect only host-plants, thus are named host-selective toxins; others affect a much broader range of hosts than the pathogen and are known as non-selective toxins (Figure 2). Blackleg of cruciferous plants is a devastating fungal disease caused by the phytopathogen Leptosphaeria maculans (Phoma lingam) and L. biglobosa. Virulent isolates of L. maculans have caused enormous yield losses in rapeseed and canola in Canada and worldwide. Recent studies of isolates of the blackleg fungus collected in different countries suggested that there are at least three distinct groups that may constitute three different species (Pedras et al., Can. J. Micro, 2007; Pedras & Yu, Nat. Prod. Commun., 2009).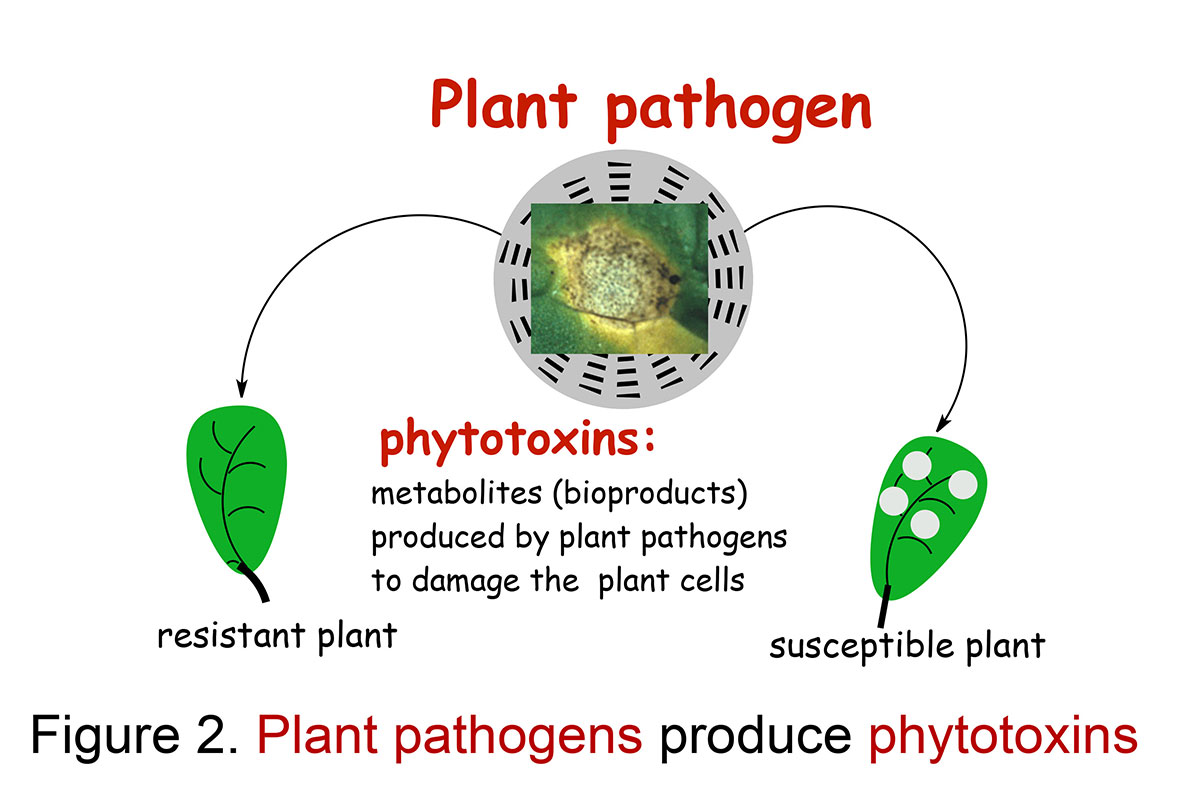
Using a bioassay-directed isolation we have discovered several types of phytotoxins produced by different isolates of L. maculans, including maculansin A, phomalide, depsilairdin, polanrazines, and phomalairdenones (Figures 1 and 3). These phytotoxins are currently being investigated to determine their role in blackleg disease of crucifers.
Alternaria blackspot is one of the most damaging plant diseases of canola, rapeseed, and mustards in Canada and also worldwide. Presently, only one type of mustard, the so-called white mustard, is resistant to this disease, but the commercial canola and rapeseed varieties are susceptible to this disease. We are trying to understand what makes white mustard resistant to this disease. Alternaria brassicae and A. brassicicola arephytopathogenic fungi that cause Alternaria black spot (also called dark leaf spot). A. brassicae produces the host-selective toxin destruxin B and related depsipeptide phytotoxins, while A. brassicicola produces brassicicolin A.
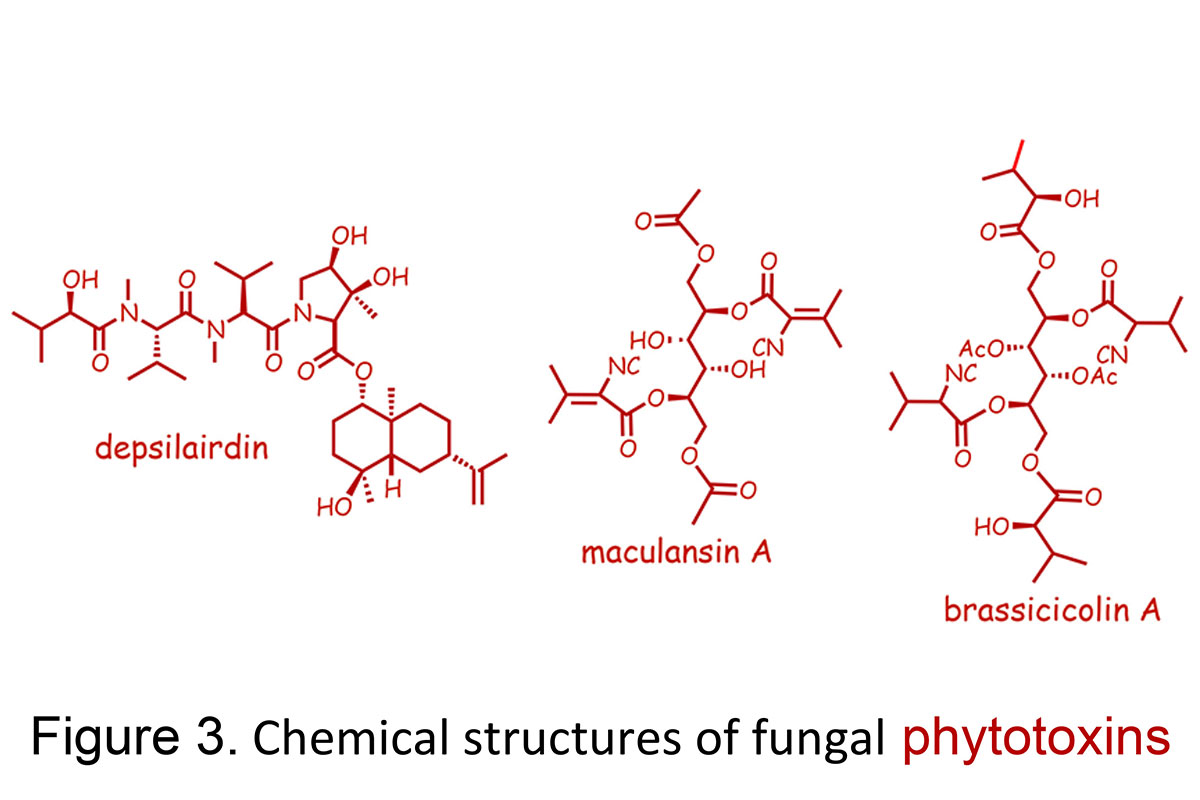
Theme 2
Synthesis and detoxification of fungal phytotoxins
Our research work on the plant disease Alternaria blackspot is an great example to demonstrate the importance of detoxification reactions (Pedras et al., Proceedings of the National Academy Sciences, USA, 2001; Phytochemistry, 2003). Work to understand what makes white mustard resistant to this disease demonstrated that white mustard leaves transformed a very phytotoxic compound, called destruxin B (14C-labeled), produced by A. brassicae, into a non-toxic compound calledhydroxydestruxin B.
The structures of hydroxydestruxin B and β-D-glucosyl hydroxydestruxin B were deduced from their spectroscopic data (NMR, HRMS, FTIR) and confirmed by total chemical synthesis (total syntheses of destruxins, collaboration with Professor Ward's team). Although these hydroxylation and glucosylation reactions occurred in both resistant (S. alba) and susceptible (Brassica napus, B. juncea, and B. rapa) species, hydroxylation was the rate limiting step in the susceptible species, whereas glucosylation was the rate limiting step in the resistant species (Figure 4). Remarkably, it was observed that hydroxydestruxin B induced the biosynthesis of phytoalexins in blackspot resistant species but not in susceptible species (Figure 5). This appears to be a unique example of phytotoxin detoxification and simultaneous phytoalexin elicitation by the detoxification product.
Our studies suggest that white mustard can overcome the fungal invader through detoxification of destruxin B coupled with production of phytoalexins (Figures 4 and 5). Since the hydroxylation reaction happens at a very fast rate and it leads to a less toxic product, it may prevent the plant cells from being killed by the pathogen. Thus, we proposed that canola plants can become resistant to the terrible blackspot disease upon incorporation of this detoxification trait. We are investigating other plant diseases utilizing host-selective toxins and similar methodology.
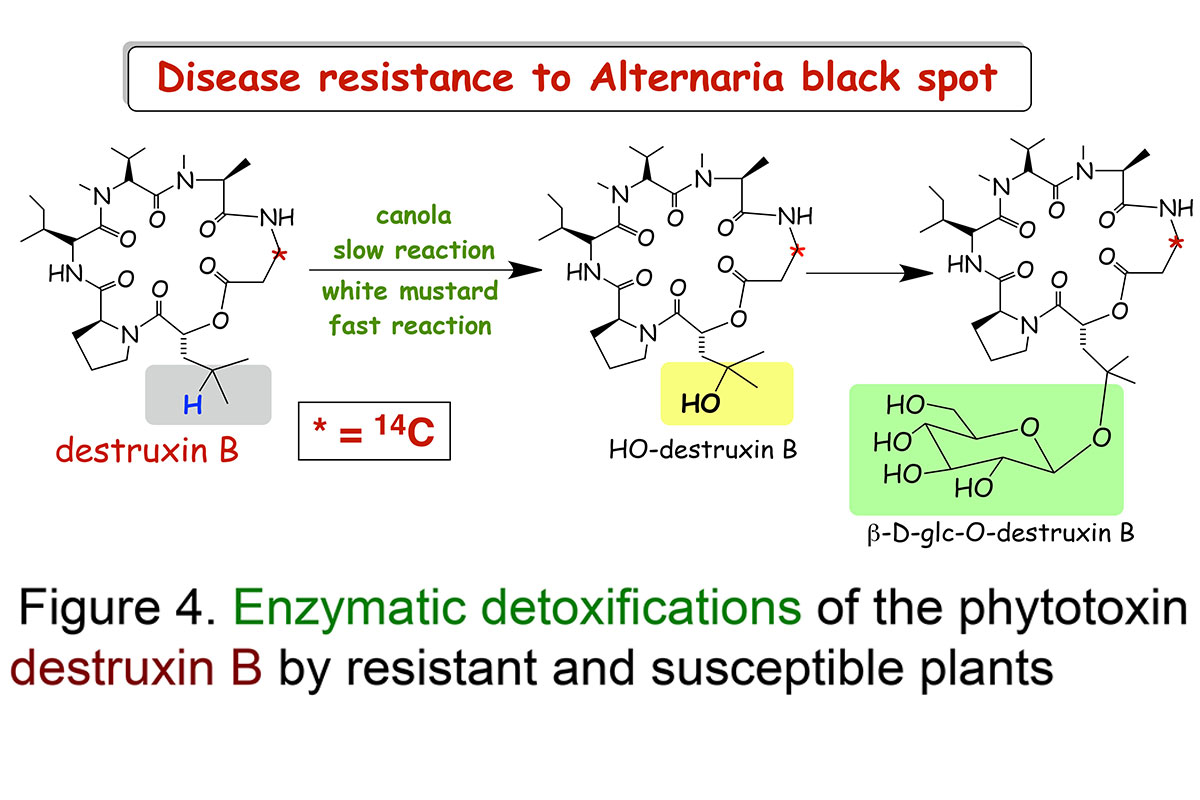
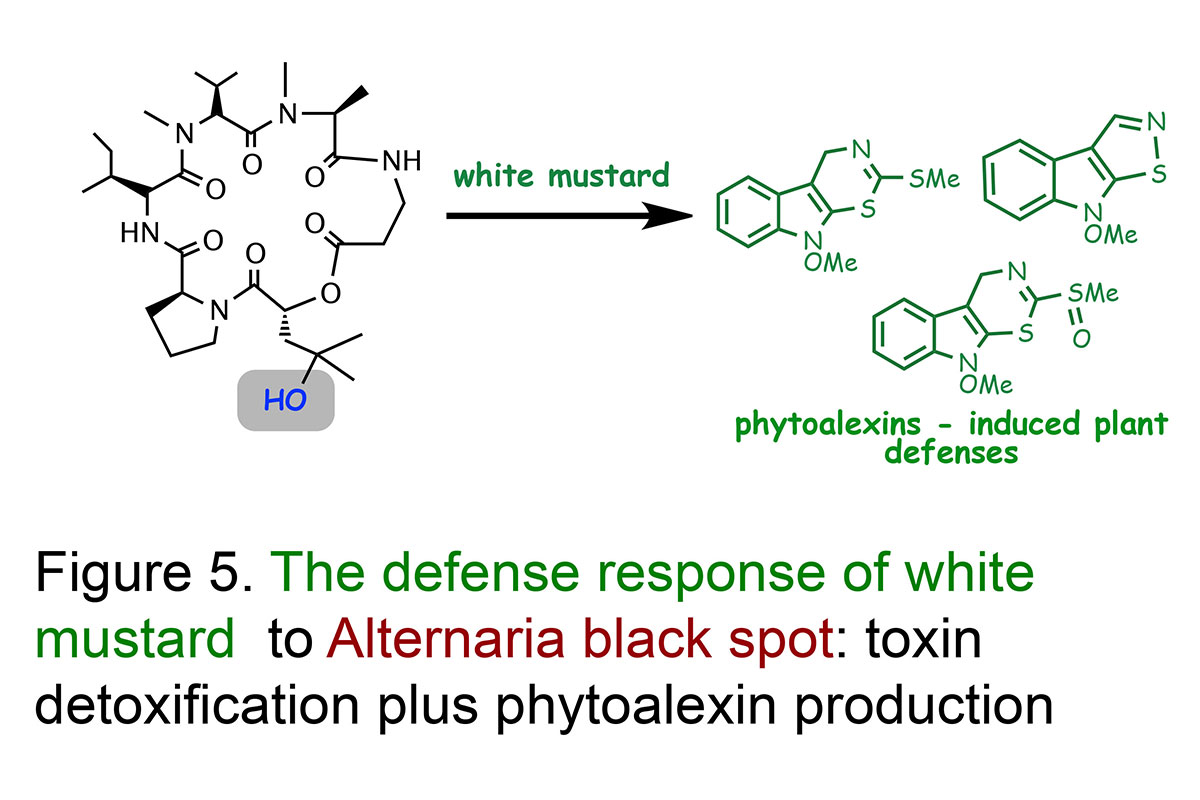
Theme 3
Isolation of phytoalexins and phytoanticipins from crucifers
Plants produce chemical defenses to protect themselves against all sorts of pests and stress, such as phytoanticipins (constitutive) and phytoalexins(inducible) (Figure 6). We have been studying the secondary metabolites of both cultivated and wild crucifers in connection with some of the main fungal diseases of these important crops. Our search for phytoalexins from cruciferous plants resistant to economically important fungal diseases lead us to examine stinkweed, a potential source of disease resistance to the virulent blackleg fungus and wasabi, a perennial plant widely cultivated in Japan, and salt cress, a wild species resistant to salt, drought and cold. Unexpectedly, we have established that wasabi, stinkweed and salt cress produce the phytoalexins wasalexins. It is anticipated that, as phytoalexins from unrelated cruciferous species are discovered the "design" of species producing "designer" phytoalexin blends active against a range of pathogens may become a feasible strategy.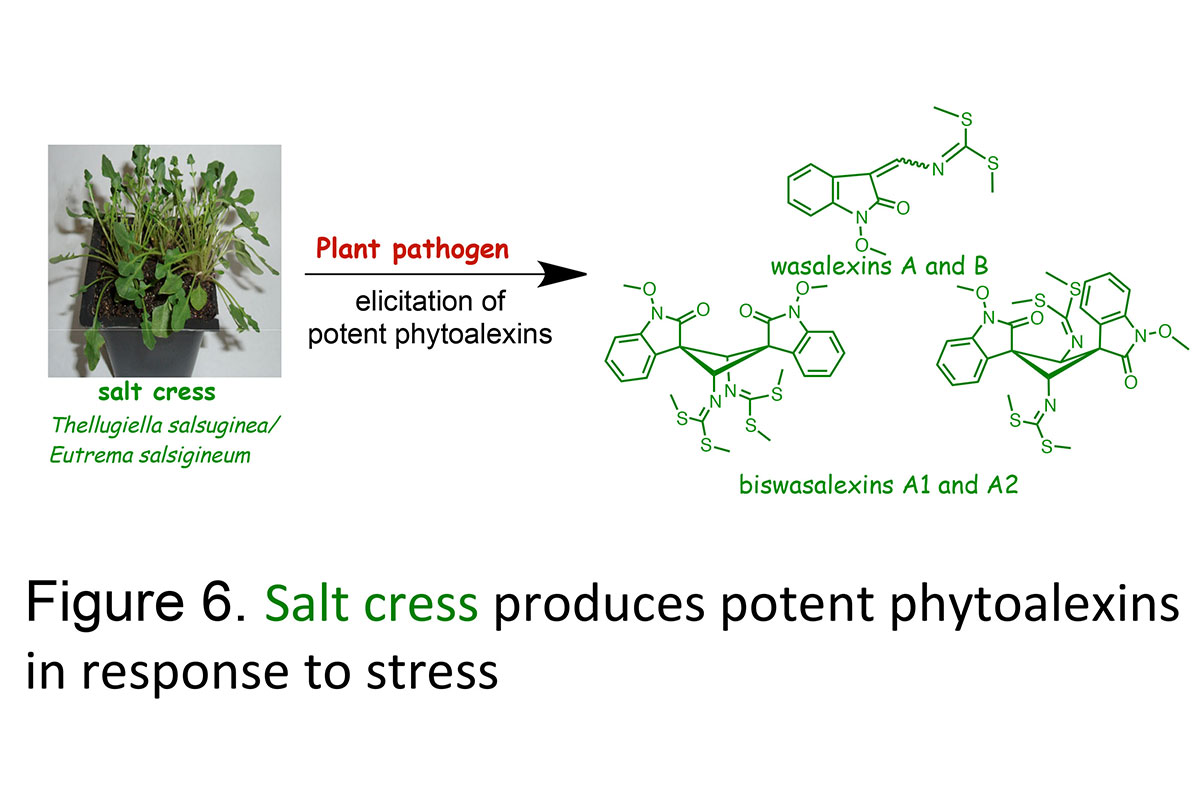
Theme 4
Biosynthesis of phytoalexins and phytotoxins
We are investigating the bio-precursors and the enzymatic processes that lead to the synthesis of phytoalexins in plants and phytotoxins in fungi. In this connection indole acetaldoxime and indole glucosinolates labelled with stable isotopes such as deuterium (D), carbon thirteen (13C), nitrogen fifteen (15N) and sulfur thirty four (34S), and their close precursors are being investigated to establish relationships among cruciferous phytoalexins, including the central role of brassinin (review: Pedras et al.,Natural Product Reports, 2011, Figure 7).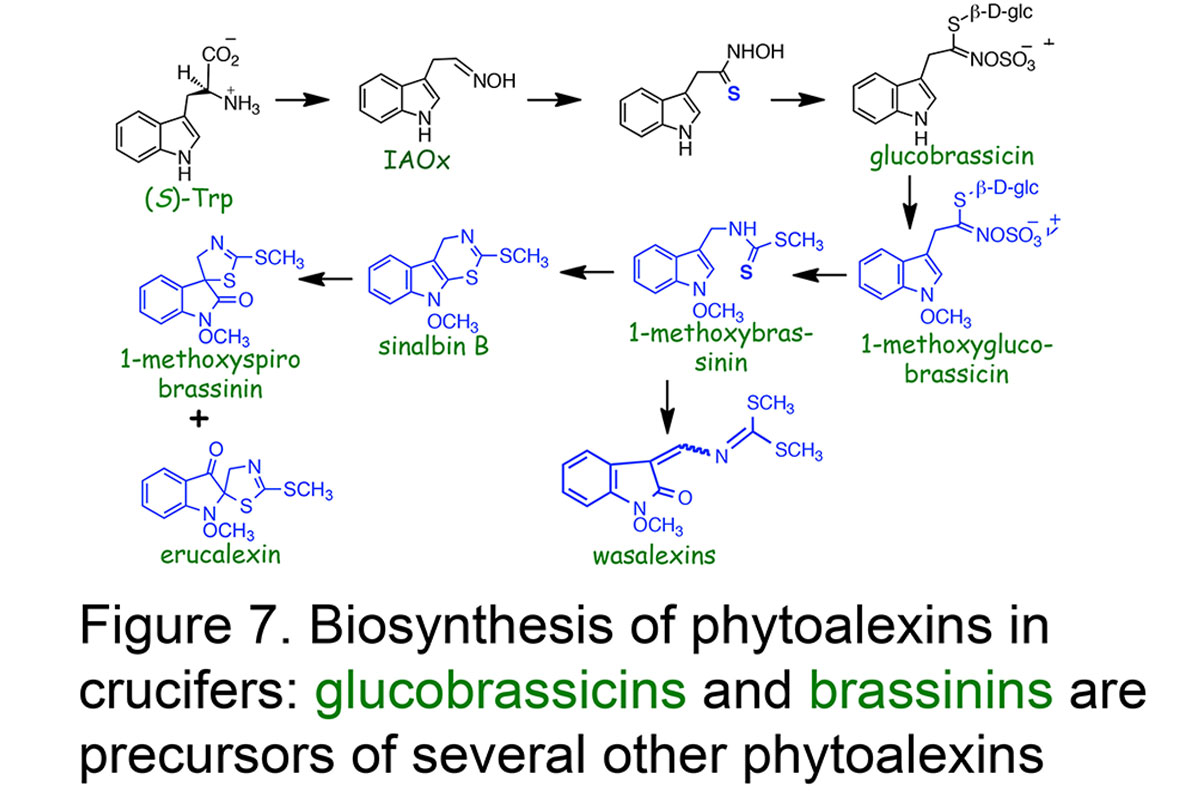
Theme 5
Synthesis & detoxification of phytoalexins and phytoanticipins
Understanding phytoalexin detoxification pathways utilized by phytopathogenic fungi and co-occurring microorganisms is of great interest to a bio-rational approach to control plant pathogens. Our studies provide remarkable examples of phytoalexin detoxification by phytopathogenic fungi, suggesting that enzymes involved in such processes may result from an intergeneric-genetic exchange between brassicas and their fungal pathogens. For example, we have shown that three different rapeseed pathogens convert the phytoalexin cyclobrassinin into three different phytoalexins, brassilexin, dioxibrassinin, and brassicanal A, and ultimately each phytoalexin into nontoxic products. We proposed that such pathogens could have acquired a more effective mechanism for overcoming this phytoalexin by "taking in" phytoalexin biosynthetic enzymes from their host plants. This strategy appears quite plausible, especially considering that most fungal pathogens have been coevolving with plants for numerous generations (Pedras & Okanga, Journal Agricultural & Food Chemistry, 1999) (Figure 8). The phytoalexin brassinin is detoxified via different pathways, which are dependent on the fungal species (review: Pedras et al.,Natural Product Reports, 2011, Figure 9).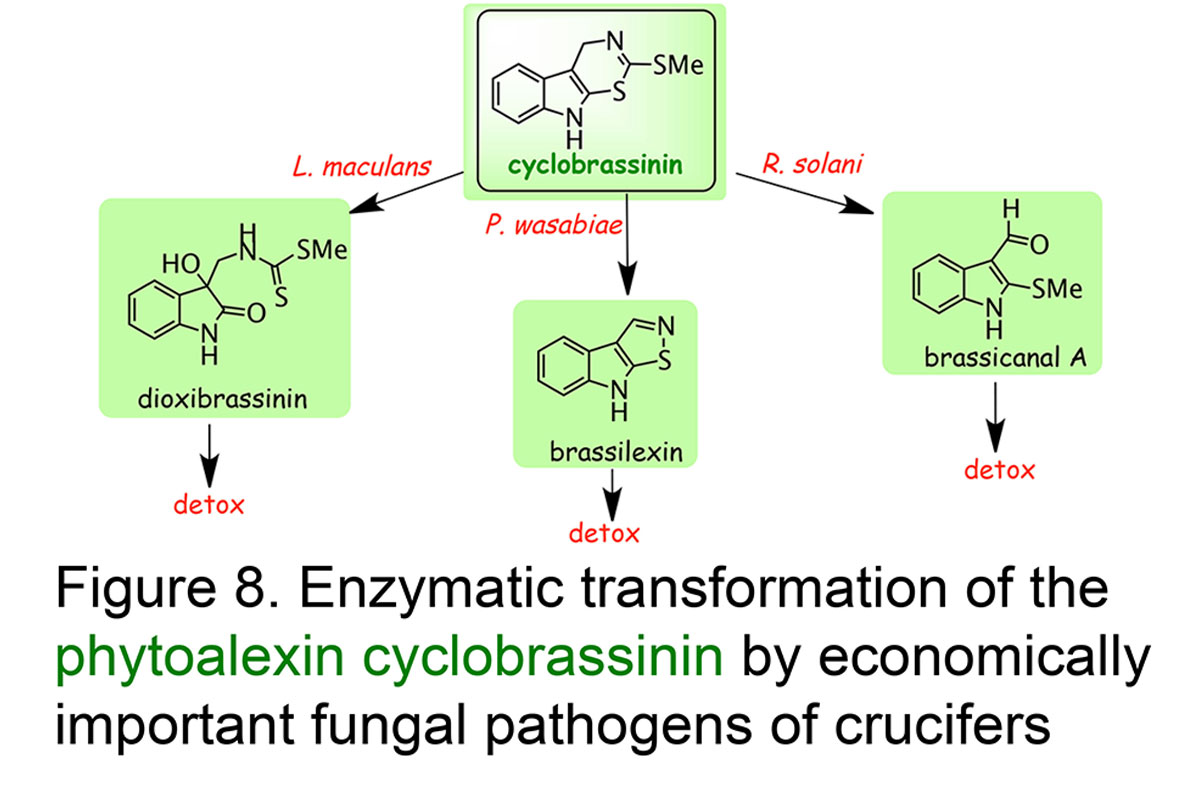
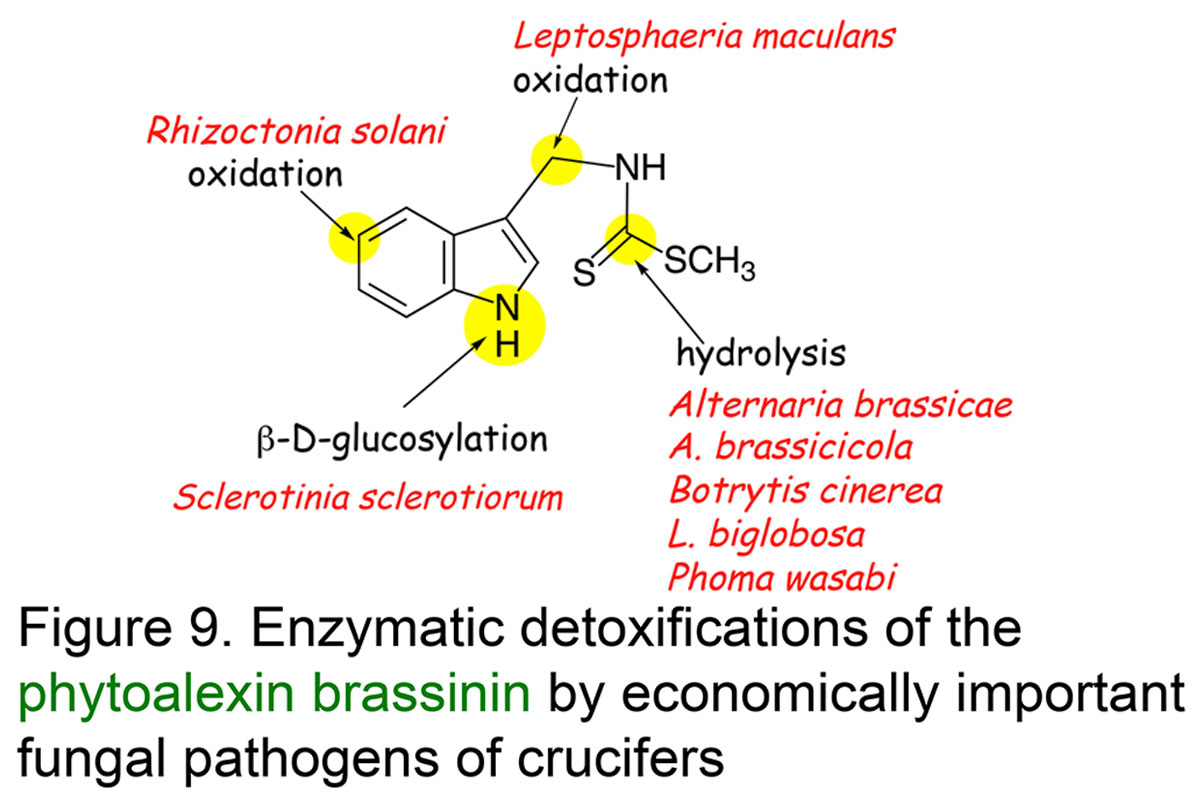
Theme 6
Isolation and characterization of phytoalexin detoxifying enzymes and synthesis of paldoxins
Plant-fungal interactions involve an enormous variety of defense and counter-attack mechanisms operating in both organisms; in many interactions successful fungal invasion of plant tissues includes production of detoxifying enzymes. Many of these fungal enzymes are involved in the detoxification of plant defenses such as phytoalexins. In an effort to create sustainable plant disease treatments, metabolic processes pertaining to specific plant fungal pathogens were searched for. Several fungal enzymes catalyzing the detoxification of phytoalexins were discovered and are currently being investigated to establish if their inhibition could protect the plant. Toward this end, fungal detoxifying enzymes were purified to homogeneity and their chemical mechanisms and inhibitors studied in detail.
To design inhibitors of phytoalexin detoxifying enzymes, i.e. paldoxins, we have been investigating the effects of various crucifer phytoalexins, their bioisosteres and other structural analogues on detoxification reactions (Fig. 10). Paldoxins are inhibitors of phytoalexin detoxification conceived to be non-toxic to living systems. Recently, fungal enzymes involved in the detoxification of a phytoalexin from canola (brassinin) were used to screen a variety of brassinin analogues and derivatives. These studies revealed structural requirements of substrates and inhibitors of brassinin detoxifying enzymes, as well as unusual mechanisms of detoxification.
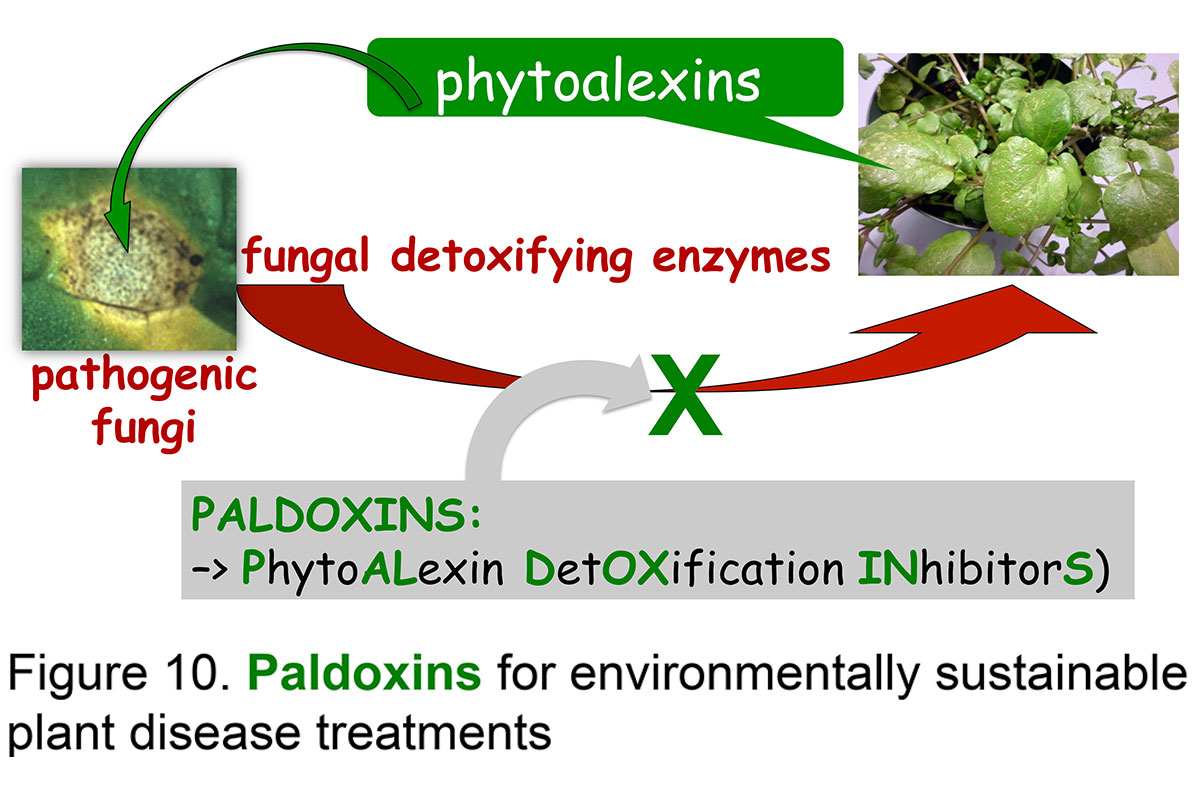